Plasma physics studies charged particles and fluids interacting with self-consistent electric and magnetic fields. It is an essential research discipline with many application areas, such as space and astrophysics, controlled fusion, accelerator physics, and beam storage. Plasma is often called the fourth state of matter, along with solid, liquid, and gas.

One of the most promising applications of plasma physics is fusion energy, which is the process of fusing light atomic nuclei into heavier ones, releasing enormous amounts of energy. Fusion energy has the potential to produce clean, safe, and abundant power for humanity without creating the dangerous radioactive waste associated with nuclear fission reactors. However, achieving fusion energy on Earth is challenging, as it requires creating and sustaining very high temperatures and pressures in a confined space.
The primary method for achieving fusion energy on Earth is using a tokamak, a doughnut-shaped chamber that uses powerful magnets to confine a plasma (a hot ionized gas) at its center. The plasma must be heated to temperatures above 100 million degrees Celsius (the Sun’s core temperature) for a long enough time to overcome the electrostatic repulsion between positively charged nuclei and allow them to fuse. Much.
The ITER project is an international collaboration that aims to build and operate the world’s largest tokamak in France. The ITER tokamak will have a diameter of 27 meters (88 feet). It will use 17 superconducting magnets to create a plasma with a temperature of over 150 million degrees Celsius (the minimum required for fusion). The ITER tokamak will also have a complex heating coils, diagnostics, and safety device system to ensure its stability and performance. The ITER project started in 2006 and is expected to be completed by 2025. Its main goal is to demonstrate that fusion can produce more energy than it consumes.
Another method for achieving fusion energy on Earth is using inertial confinement (ICF), which involves compressing a small amount of fuel (usually deuterium or tritium) into a tiny sphere using powerful lasers or particle beams. The sphere then implodes under its own gravity or shock wave, creating high temperatures and pressures that can trigger fusion reactions. , such as achieving high confinement times, densities, and temperatures for sustained fusion reactions.
The National Ignition Facility (NIF) in California is an example of an ICF facility that uses 192 powerful lasers to compress gold-plated fuel pellets into microscopic spheres. The NIF achieved a significant milestone in June 2023 when it reported extracting more energy from a controlled nuclear fusion reaction than had been used to trigger it. This was considered a global first and a significant step for physics research on fusion.
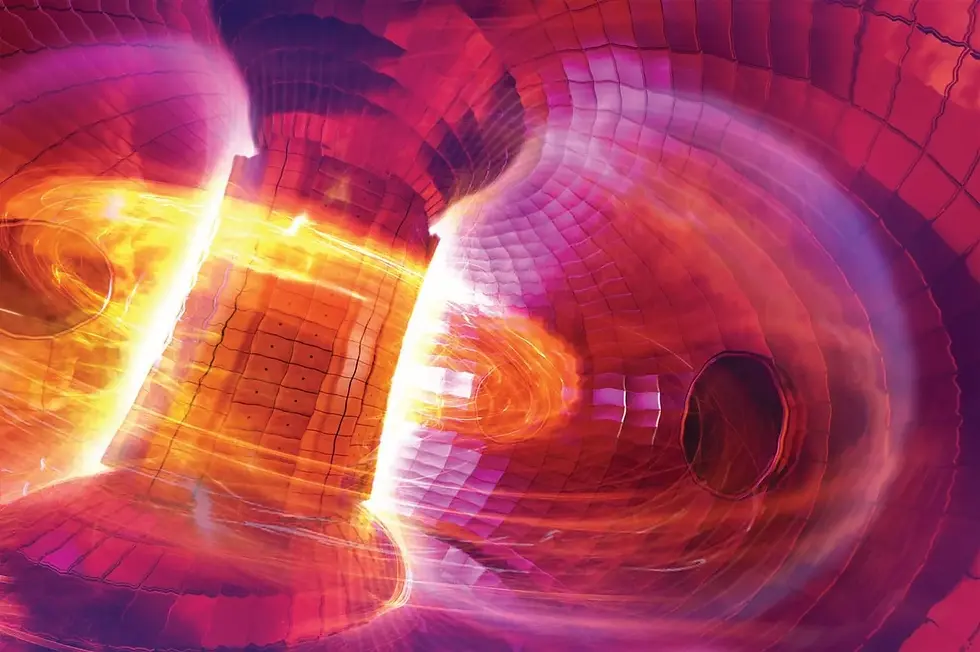
Plasma physics may hold the key to our energy needs through fusion energy machines because it offers several advantages over other forms of energy production. Some of these advantages are:
Fusion energy produces no greenhouse gases or air pollutants contributing to climate change or environmental degradation.
Fusion energy uses abundant fuels such as hydrogen isotopes that can be extracted from water or mined from asteroids.
Fusion energy does not generate radioactive waste or pose proliferation risks due to its low-energy output.
Fusion energy has a virtually unlimited fuel supply compared to fossil fuels or nuclear fission reactors.
Fusion energy has high efficiency and reliability due to its self-sufficient nature.
However, plasma physics faces many challenges and uncertainties before becoming a viable source of large-scale power for our planet. Some of these challenges are:
Achieving stable and sustained fusion reactions at high temperatures and pressures requires overcoming physical obstacles such as plasma instabilities, turbulence, confinement time limitations, heat loss mechanisms, etc.
Developing safe and robust materials that can withstand extreme conditions such as radiation exposure, neutron bombardment, etc.
Scaling up existing technologies, such as tokamaks or ICFs, from laboratory prototypes to industrial plants without compromising their performance or safety.
Reducing costs and increasing public acceptance of fusion technology due to its long-term nature.
Therefore, plasma physics remains an active area of research that requires further innovation and collaboration among scientists from different disciplines such as physics, engineering, chemistry, etc., as well as stakeholders from industry, government, etc., who can provide funding support, policies, guidance, etc., for its development.
Comments